Review Article, Int J Ophthalmic Pathol Vol: 12 Issue: 1
The Blood Ocular Barrier: An Anatomical Review
Jeremy Williamson*
Department of Colorectal Surgery, Royal Gwent Hospital, Newport, United Kingdom
*Corresponding Author: Jeremy Williamson
Department of Colorectal Surgery, Royal Gwent Hospital, Newport, United Kingdom; Tel: 403844892;
E-mail: jeremypwilliamson@gmail.com
Received date: 14 January, 2023, Manuscript No. IOPJ-23-32019;
Editor assigned date: 18 January, 2023, PreQC No. IOPJ-23-32019 (PQ);
Reviewed date: 01 February, 2023, QC No. IOPJ-23-32019;
Revised date: 08 February, 2023, Manuscript No. IOPJ-23-32019 (R);
Published date: 15 February, 2023, DOI: 10.4172/2324-8599.12.1.115
Citation:Williamson J (2023) The Blood Ocular Barrier: An Anatomical Review. Int J Ophthalmic Pathol 12:1.
Abstract
The blood ocular barriers serve critical functions in maintaining physiological conditions in the human eye. The blood ocular barrier is primarily divided into two components, the blood aqueous barrier and the blood retinal barrier, itself divisible into the inner and outer blood retinal barrier. The existences of such barriers were postulated following the discovery of the blood brain barrier in the late 19th century. Understanding of the blood barrier was in part due to experiments in which trypan blue was injected intravenously into the circulation of animals resulting in bright blue staining of the tissues of the animal’s body globally with the exception of the brain and spinal cord, which was described as snow white. Further derivatives of these experiments lead to the discovery of the blood aqueous barrier. The blood retinal barrier was subsequently discovered by studying the effect of histamine on blood vessels around the body. Peripherally histamine increases permeability of blood vessels however cerebral and retinal vessels are unique in this way, as histamine has no effect on their permeability.
Keywords: Blood ocular barriers, Blood brain barrier, Human eye, Histamine
Description
The blood ocular barriers of the body can be damaged due to a variety of insults including trauma, local or systemic disease. This damage can be sight limiting and remains a challenge in management of patients with these conditions today. This review will discuss the microanatomy involved in these barriers in depth with a broader discussion of the sequalae of damage to these barriers thereafter [1].
Literature Review
Blood aqueous barrier
The blood Aqueous Barrier (BAB) was the first of the two known blood ocular barriers discovered. The BAB may be better described as a series of barriers which together regulate substances entering the aqueous humor from the plasma [2]. Individual structures which together form the blood aqueous barrier include the non-pigmented ciliary epithelium of the ciliary body, the posterior iris epithelium, the endothelium of the iris vasculature and the inner wall endothelium of Schlemm’s canal. These structures are represented graphically in Figure 1.
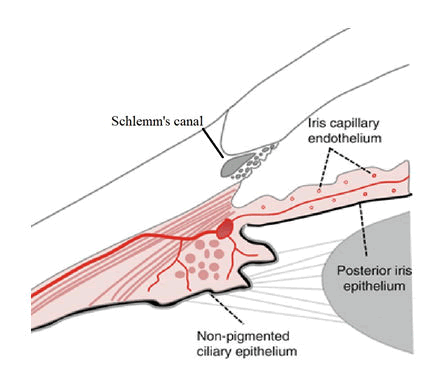
Figure 1: Anatomical structures involved in the BAB adapted from ocular circulation.
Discussion
The ciliary body forms part of the uveal tract and divides the anterior and posterior segments of the eye. The ciliary body is triangular in cross section with apex pointing posteriorly toward the ora serrata and base attaching to the sclera. The most anterior attachments are at the scleral spur, the anterior side of the triangle joins the iris. This structure has small projections called ciliary processes. The ciliary processes, 60-80 in number are the site of aqueous production [3]. The ciliary processes are covered in a double layer of epithelium. This epithelium is continuous with the posterior iris and hence forms a continuous barrier. The two epithelial layers are the pigmented epithelium followed by the non pigmented epithelium. The epithelial cells comprising these layers are packed tightly together, with their apical surfaces abutting. The abutting surfaces between these cells are joined by tight junctions, or zonulae occludentes; sourced from basic and clinical science course, AAO. This epithelium is impermeable to larger molecules, particularly proteins.
The ciliary body and it’s uveal neighbour the iris, form from the optic cup in utero. The inner and outer layer of the optic cup which will in turn become the non-pigmented epithelium and the pigmented epithelium respectively begin to form ridges towards the end of the third month of gestation. These ridges will in turn become the ciliary processes. Around this time the neuroectoderm, which is continuous with this area and toward the terminal tips of the optic cup begins to stretch and grow between the lens and developing cornea forming the iris. Similarly, to the ciliary processes the inner and outer layer of the optic cup becomes the posterior and anterior iris epithelium respectively. It is also during the third month that zonulae occludentes are first seen [4]. Literature suggests these zonulae occludentes which consist of branching networks of sealing strands have relatively fewer sealing strands than those involved in the blood retinal barrier and therefore create a slightly more permeable barrier in comparison. They are therefore labelled zonulae occludentes of the ‘leaky’ type. These junctions are more permeable to molecules with greater lipid solubility.
Iris blood vessels also have zonulae occludentes and lack fenestrations which similarly makes them largely impermeable to larger molecules. These capillaries are arranged in coils within the iris stromal tissues which allows for dilation of the iris whilst keeping the zonulae occludentes patent. The final structure known to be involved in the blood aqueous barrier is the endothelium lining Schlemm’s canal. This critical final interface between aqueous and blood-in this instance from the episcleral veins, must allow outflow of aqueous whilst maintaining the BAB. There is not yet a clear consensus on how Schlemm’s canal can act as an outflow tract whilst maintaining the BAB. However some models suggest micro pores combined with an oncotic pressure gradient enable this largely one way flow (Figure 2) [5].
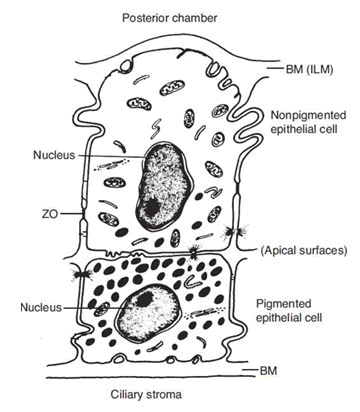
Figure 2: The pigmented and non-pigmented ciliary epithelium. Note: Basement Membrane (BM) lines the double layer and comprises the Internal Limiting Membrane (ILM) on the inner surface. The abutting edges of the nonpigmented epithelium is sealed by Zonula Occludentes (ZO). Adapted from basic and clinical science course, AAO
These barriers rely on ocular pressure to remain patent. Ocular hypotony (or ocular injury/disease) can cause the opening of the nonfenestrated endothelial layer of the iris capillaries and the opening of the zonulae occludentes of the ciliary body. Creating an influx of molecules into the aqueous that are not present in a physiological state [6].
These barriers when operating physiologically blocks proteins from entering the aqueous which allows for a near protein free environment (approximately 0.5% of plasma protein concentration) in the anterior the blood aqueous barrier is also able to maintain proportionally higher ascorbate acid levels, slightly higher electrolyte levels, higher concentrations of certain amino acids and far lower levels of immune globulins than plasma, the latter of which markedly increases in conditions such as intraocular infection or uveitis. Glucose is also present in the aqueous, approximately half the concentration of serum, however this can increase in diabetes. This tightly controlled aqueous composition is required to nourish, maintain intraocular pressure, maintain immune privilege as well as maintain visual acuity. Aqueous has a refractive index of 1.336, even lower than the cornea allowing transmission of light with minimal chromatic aberration [7].
Blood Retinal Barrier (BRB)
The blood retinal barrier is located in the posterior segment of the eye and is comprised of two parts, the inner Blood Retinal Barrier (iBRB) and the outer Blood Retinal Barrier (oBRB). The iBRB consists of the inner retinal microvascular endothelium and specialised pericytes and muller cells whereas the oBRB consists of the retinal pigment epithelial cell layer creating a barrier between the choroid and the sub-retinal space. The schematic in Figure 3 shows the locations of the iBRB and oBRB in relation to the cross sectional wall of the retina and the choroid.
As in the BAB, both the iBRB and oBRB limit paracellular transport of molecules by zonulae occludentes however they are of the nonleaky type. Embryological formation of the iBRB and oBRB is discussed below separately but develops in the context of retinal development. The neuro retina is formed when the inner layer of the optic cup is signalled by overlying ectoderm to differentiate into the neural retina [8]. The retinal vasculature develops from the optic disc and radiates circumferentially to cover the retina. This vasculature grows and continues to branch, eventually forming vascular buds at the tips of the vessels. These vessels radiate and differentiate into arteries and veins (Figure 3).
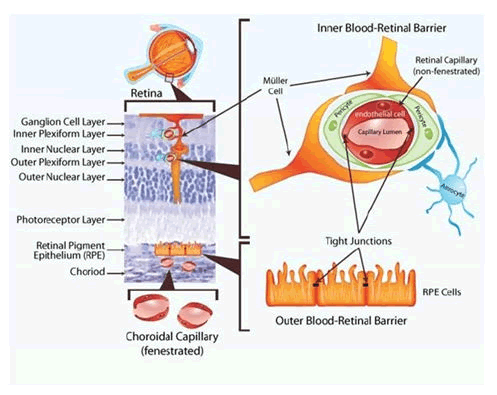
Figure 3: Inner and outer blood retinal barriers.
Inner blood retinal barrier
The endothelium lining the retinal capillaries are not fenestrated like peripheral capillaries and have few vesicles. This endothelium forms the central part of the iBRB and the junctions of the individual endothelial cells have zonulae occludentes or tight junctions. The endothelium is then encircled by pericytes which themselves are lined exteriorly by foot processes of astrocytes and muller cells. This schematic also demonstrates the two layers the superficial and deep capillary plexuses are located within. The superficial within the inner plexiform or ganglion cell later and the deep within the junction between the inner nuclear layer and the outer plexiform layer [9].
The pericytes in the retina are more densely packed than in any other capillary bed; they serve many important purposes including regulating vascular tone by contracting and releasing contractile proteins, providing structural support to the vascular wall, inhibiting endothelial cell proliferation and are phagocytotic. Muller cells are the primary glial of the retina and play a key role in modulating the neuronal activity of the retina by regulating nutrient uptake, neurotransmission, electrolyte homeostasis and regulating the secretion of Vascular Endothelial Growth Factor (VEGF) [10]. Astrocytes which are the final key cell in the iBRB play an important role. They upregulate the secretion of several specialised proteins such as occludin, claudin-5 and ZO-1. These proteins are all building blocks of the zonulae occludentes and are represented diagrammatically in Figure 4.
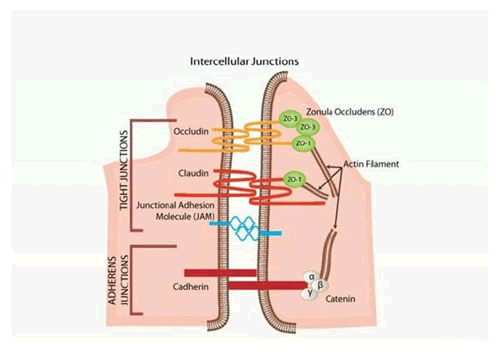
Figure 4: Intercellular junctions.
Mice models have been used to study the embryological formation of the iBRB, collagen IV which is present in the retinal vascular basal lamina can be stained using anti-collagen antibodies and retinal astrocytes can be stained using anti-glial antibodies. In mouse models, on the day of birth no capillary retinal vessels were observed on electron microscopy however at postnatal day 3, preliminary capillaries were observed in the ganglion layer, the endothelium and astrocytes appeared to form simultaneously, suggesting a shared role in development and the first formation of an immature iBRB at this time. By day 5 of life zonulae occludentes can be seen [11].
Outer blood retinal barrier
In utero after the neural retina is formed, the outer layer with interaction from the surrounding extra ocular mesenchyme differentiates into the Retinal Pigmented Epithelium (RPE). This is the first retinal layer to differentiate. It is pigmented at approximately week 3 or 4 of gestation and is the first pigmentation that occurs in the human embryo. After week 6 the RPE is one cell thick. This monolayer of cells performs many functions, not the least of which is the oBRB.
The oBRB comprises the RPE and the zonulae occludentes that seal the interface of the cells. This layer sits between the photoreceptor cells and the choroidal vasculature. The oBRB also serves as a boundary between the fenestrated capillaries of the choroid and the non-fenestrated capillaries of the inner retina. Transcellular and paracellular transport of the blood components to the retina does occur at the oBRB but it is tightly regulated. Electrolytes and water also permeate in strictly controlled conditions. Outward transport of waste products from the retina also occurs here and is removed from the area by the chorio capillaris [12].
Supplementary barrier mechanisms
The internal limiting membrane of both the anterior and posterior segments appears to offer some resistance to the passage of molecules from the blood into the respective ocular segments. In the anterior chamber the uveoscleral or unconventional pathway of aqueous drainage provides another potential interface between blood and aqueous, the inflows from this tract are however not well understood. Posteriorly the relatively dense, type II collagen matrix present in the cortical vitreous also provides some resistance to the diffusion of molecules. Uniquely Bruch’s membrane has been shown to have decreasing permeability as age increases. Therefore at an advanced age it can be considered a supplementary barrier to the oBRB [13].
Breakdown of the blood ocular barriers
Permeability of the blood ocular barriers can increase under certain conditions, some mechanisms are common to the BAB, iBRB and oBRB and some are distinct. Any breakdown may lead to loss or degradation of vision. The BAB can have increased permeability due to biochemical mediators, such as when exposed to prostaglandins and neural peptides, such as substance P. As well as when exposed to certain mechanical/structural conditions such as hypotony, intraocular infections, topically applied drugs, inflammatory conditions particularly uveitis or trauma from corneal abrasions or surgery [14]. When the BAB is dysfunctional protein can flood into the aqueous having disastrous effects on visual acuity and depending on the cause, redness and pain. Long term sequalae of cataracts, glaucoma, and posterior segment complications can occur depending on the cause.
Damage to the iBRB most commonly occurs secondary to retinal hypoxia. The mechanism by which this occurs is complex however under hypoxic conditions VEGF secretion is increased. This has been shown to be potentiated by both the muller cells and the astrocytes in both hypoxic and inflammatory conditions. VEGF increases vascular permeability and is also pro inflammatory increasing leukocyte adhesion to vascular endothelium. In addition to VEGF, Nitric Oxide (NO) has been shown to be increased in hypoxic conditions, having vasodilatory effects and increasing blood flow which further breaks down the iBRB. The sequalae of this vasodilation is vasogenic oedema, tissue damage and results in visual loss.
The most common cause of iBRB breakdown is diabetes, this appears to be due to a range of factors early in the disease course capillary basement membrane thickens, vasodilation occurs and pericyte density is reduced. Occludin, required for the construction of zonulae occludentes is reduced, which causes increased vascular permeability. These factors combine to cause an increase in extracellular fluid in the retina, particularly the macula which may manifest in cystoid macular oedema. Naturally, direct ischaemic insult to the retina from ischaemic central retinal vein occlusion, will also cause iBRB breakdown [15].
Damage to the oBRB is less well understood than the iBRB. However evidence of oBRB damage in both ischaemic conditions and in diabetes is increasing in the literature. In addition to these conditions, chronic oxidative stress is reported to be a major factor in oBRB is certain conditions, such as in Age Related Macular Degeneration (ARMD). The oBRB also has increased permeability in chronic retinal detachment likely due to decreased metabolism secondary to retinal neuronal death. The oBRB is also vulnerable to severe insults to the choroidal circulation, this can occur in end stage accelerated hypertension, toxaemia of pregnancy, or choroidal malignancy. The sequalae of the breakdown of the oBRB (the RPE) is serous retinal detachment.
Conclusion
The blood ocular barrier can be divided into the blood aqueous barrier in the anterior segment and the blood retinal barrier in the posterior segment, the latter is further divisible into the inner and outer blood retinal barrier. These barriers ensure the anterior and posterior segments of the eye are kept in a physiological state, immune privileged, with correct pressures and distribution of fluid to maintain visual acuity and ocular health. The aetiology of blood ocular barrier breakdown is numerous however inflammation, infection or trauma are most commonly implicated in breakdown of the blood aqueous barrier and diabetic retinopathy and ischaemia are the most common causes for breakdown of the blood retinal barrier. All of which are sight threatening.
References
- Cunha Vaz JG (1997) The blood ocular barriers: Past, present and future. Doc Ophthalmol 93: 149-157.
[Crossref] [Google Scholar] [PubMed] - Occhiutto ML, Freitas FR, Maranhao RC, Costa VP (2012) Breakdown of the blood ocular barrier as a strategy for the systemic use of nanosystems. Pharmaceu 4: 252-275.
[Crossref] [Google Scholar] - Skalicky SE (2016) Ocular and visual physiology. Clinical Application, 1st edition, Springer Singapore, Singapore, pp. 167-179.
[Google Scholar] - Jacob S (2008) Chapter 7-Head and neck. Churchill Livingstone: London, UK, pp. 181-225.
[Crossref] [Google Scholar] - Remington LA (2012) Chapter 7-Ocular embryology. Clinical Anatomy and Physiology of the Visual System, 3rd edition, Saint Louis: Butterworth-Heinemann, Oregon, pp. 123-143.
[Crossref] - Caileanu GD, Stanila A (2017) Aqueous humor: Physiology and dynamics. Acta Medica Transilvanica 22: 54-56.
[Google Scholar] - Forrester JV (2016) Iris Blood Vessel-An overview. 4th edition, Aberdeen.
- Braakman ST, Moore Jr JE, Ethier CR, Overby DR (2016) Transport across Schlemm's canal endothelium and the blood aqueous barrier. Exp Eye Res 146: 17-21.
[Crossref] [Google Scholar] [PubMed] - Campbell M, Humphries P (2013) The blood retina barrier: Tight junctions and barrier modulation. Adv Exp Med Biol 763: 70-84.
[Crossref] [Google Scholar] [PubMed] - Fuhrmann S, Zou C, Levine EM (2014) Retinal pigment epithelium development, plasticity and tissue homeostasis. Exp Eye Res 123: 141-150.
[Crossref] [Google Scholar] [PubMed] - AAO (2019) Basic and Clinical Science Course, Section 09, 2019-2020: Uveitis and Ocular Inflammation, American Academy of Ophthalmology, pp. 384.
- Yao H, Wang T, Deng J, Liu D, Li X, et al. (2014) The development of blood retinal barrier during the interaction of astrocytes with vascular wall cells. Neu Reg Res 9: 1047.
[Crossref] [Google Scholar] [PubMed] - Yang AH, Huang W (2012) Retinal vein occlusion induced by a MEK inhibitor-Impact of oxidative stress on the blood-retinal barrier. Oxidative Stress and Diseases, USA, pp. 469-494.
[Google Scholar] - Kaur C, Foulds WS, Ling EA (2008) Blood retinal barrier in hypoxic ischaemic conditions: Basic concepts, clinical features and management. Progr Retin Eye Res 27: 622-647.
[Crossref] [Google Scholar] [PubMed] - Newman E, Reichenbach A (1996) The müller cell: A functional element of the retina. Trends Neurosci 19: 307-312.
[Crossref] [Google Scholar] [PubMed] - van der Wijk AE, Wisniewska-Kruk J, Vogels IM, van Veen HA, Ip WF, et al. (2019) Expression patterns of endothelial permeability pathways in the development of the blood-retinal barrier in mice. FASEB J 33: 5320-5333.
[Crossref] [Google Scholar] [PubMed] - Johnson M, McLaren JW, Overby DR (2017) Unconventional aqueous humor outflow: A review. Exp Eye Res 158: 94-111.
[Crossref] [Google Scholar] [PubMed] - Moore DJ, Hussain AA, Marshall J (1995) Age related variation in the hydraulic conductivity of Bruch's membrane. Inv Ophthalmol Vis Sci 36: 1290-1297.
[Google Scholar] [PubMed] - Stamper RL, Lieberman MF, Drake MV (2009) CHAPTER 2-Aqueous humor formation. Becker-Shaffer's diagnosis and therapy of the glaucomas E-Book, 8th edition, Elsevier Health Sciences, US, pp. 8-24.
[Crossref] [Google Scholar] - Menezo V, Lightman S (2005) The development of complications in patients with chronic anterior uveitis. Ame J Ophthalmol 139: 988-992.
[Crossref] [Google Scholar] [PubMed] - Schoch HJ, Fischer S, Marti HH (2002) Hypoxia induced vascular endothelial growth factor expression causes vascular leakage in the brain. Brain 125: 2549-2557.
[Crossref] [Google Scholar] - Rudraraju M, Narayanan SP, Somanath PR (2020) Regulation of blood retinal barrier cell junctions in diabetic retinopathy. Pharmacol Res 161: 105115.
[Crossref] [Google Scholar] [PubMed] - Eichler W, Kuhrt H, Hoffmann S, Wiedemann P, Reichenbach A (2000) VEGF release by retinal glia depends on both oxygen and glucose supply. Neuroreport 11: 3533-3537.
[Crossref] [Google Scholar] [PubMed] - Kaur C, Sivakumar V, Foulds WS (2006) Early response of neurons and glial cells to hypoxia in the retina. Invest Ophthalmol Vis Sci 47: 1126-1141.
[Crossref] [Google Scholar] [PubMed] - Joussen AM, Poulaki V, Qin W, Kirchhof B, Mitsiades N, et al. (2002) Retinal vascular endothelial growth factor induces intercellular adhesion molecule-1 and endothelial nitric oxide synthase expression and initiates early diabetic retinal leukocyte adhesion in vivo. Am J Pathol 160: 501-509.
[Crossref] [Google Scholar] [PubMed] - Barber AJ, Antonetti DA, Gardner TW (2000) Altered expression of retinal occludin and glial fibrillary acidic protein in experimental diabetes. Invest Ophthalmol Vis Sci 41: 3561-3568.
[Google Scholar] [PubMed] - Cunha-Vaz J, Bernardes R, Lobo C (2011) Blood-retinal barrier. Eur J Ophthalmol 21: 3-9.
[Crossref] [Google Scholar] [PubMed] - Xia T, Rizzolo LJ (2017) Effects of diabetic retinopathy on the barrier functions of the retinal pigment epithelium. Vision Res 139: 72-81.
[Crossref] [Google Scholar] [PubMed] - Xu HZ, Le YZ (2011) Significance of outer blood-retina barrier breakdown in diabetes and ischemia. Invest Ophthalmol Vis Sci 52: 2160-2164.
[Crossref] [Google Scholar] [PubMed] - Bailey TA, Kanuga N, Romero IA, Greenwood J, Luthert PJ, et al. (2004) Oxidative stress affects the junctional integrity of retinal pigment epithelial cells. Invest Ophthalmol Vis Sci 45: 675-684.
[Crossref] [Google Scholar] [PubMed] - Soubrane G, Coscas G (2013) Chapter 30-Pathogenesis of serous detachment of the retina and pigment epithelium. Retina, 5th edition, Elsevier Health Sciences, US, pp. 618-623.
[Crossref]