Research Article, Jrgm Vol: 9 Issue: 1
Regenerative Surgery in Open Mesh Repair for Inguinal Hernia
Valerio Di Nicola*
West Sussex Hospitals NHS Foundation Trust, Worthing Hospital, BN112DH, United Kingdom
*Corresponding Author: Valerio Di Nicola Regenerative Surgery Unit, Villa Aurora Hospital, Worthing Hospital, BN112DH, United Kingdom, Tel: +447557904032, E-mail: dinicolavalerio@gmail.com
Received: March 07, 2020 Accepted: April 23, 2020 Published: April 30, 2020
Citation: Nicola VD (2020) Regenerative Surgery in Open Mesh Repair for Inguinal Hernia. J Regen Med 9:1.
Abstract
Inguinal hernia repair is the most frequently performed operation in General Surgery. Over 20 million hernia repair procedures per year are performed worldwide. Complications such as chronic inguinal pain (12%) and recurrence rate (11%) significantly impact the surgical results. The 4 main impacting factors affecting hernia repair results are: mesh material and design; mesh fixation; tissue healing and regeneration and the surgical technique. These essential components are analysed in this article. This analysis is followed by an explanation of how a new procedure, L-PRF-Open Mesh Repair, can improve both short and long term results.
Keywords: Tissue regeneration, Hernia repair, Wound healing, Mesh, Mesh fixation
Keywords
Tissue regeneration, Hernia repair, Wound healing, Mesh, Mesh fixation
Introduction
Inguinal hernia open mesh repair is one of the most frequently performed surgical procedures. Literature indicates over 20 million hernia repair operations per year worldwide [4]. The number of procedures has been increasing and is envisaged to further increase due to several risk factors including chronic disease such as COPD, obesity and sedentary lifestyle [5].
The use of hernia mesh products to surgically repair or reconstruct anatomical defects has been adopted and developed over the last 30 years. The surgical mesh definitely helps to close the abdominal wall defects and to reinforce the weakened area through the incorporation of fibro-collagenous tissue. A “tension-free” repair with mesh is associated with less postoperative pain and faster recovery [6].
Open inguinal hernia repair is the most common technique and is performed by making an incision in the inguinal region by way of the subcutaneous tissue and the anterior fascia, and then the sac is identified and dissected from the cord/round ligament. Once the hernia sac is divided from the adjacent structures and examined for contents, this is pushed back into the peritoneal cavity, and finally, hernia repair is carried out.
Historically the repair has been executed in two ways
(1) Primary repair such as Bassini or Shouldice techniques, involves the sewing of the abdominal wall layers using sutures. This method is considered obsolete nowadays and has, in the last decades, been replaced by (2) patch or mesh repair. The last relies on the placement of a mesh to seal the hernia defect and reinforce the surrounding tissue. The Lichtenstein technique requires stitches to fix the located mesh whereas other procedures fix it using either fibrin or cyanoacrylate glue [1]. The results of hernia surgery are mainly measured by postoperative pain, time to return to work, short-term complications, chronic groin pain, and hernia recurrence.
In 2002, the European Union Hernia Trialists Collaboration, a group of surgical trialists who have participated in randomized trials of open mesh or laparoscopic groin hernia repair, analysed 58 randomized controlled trials and concluded that the use of surgical meshes was superior to any other techniques [7]. In particular, they noted fewer recurrences and less postoperative pain with mesh repair.
The expected rate of recurrence following inguinal hernia repair is currently 11%. Only 57% of all inguinal hernia recurrences occurred within 10 years after the hernia operation. Some of the remaining 43% of all recurrences happened only much later, even after more than 50 years [8]. A further complication after inguinal hernia repair is chronic groin pain lasting more than 3 months, occurring in 10-12% of all patients. Approximately 1-6% of patients have severe chronic pain with long-term disability, thus requiring treatment [9]
In their International Guidelines, the Hernia Surge Group strongly recommends a mesh- based repair technique for patients with inguinal and/or femoral hernia [9]. The new International Guidelines of the Hernia Surge Group recommend only the open Lichtenstein mesh technique and the laparoscopic mesh procedures TEP and TAPP as repair techniques in inguinal hernia surgery [10].
Two meta-analyses comparing only TEP and one comparing only TAPP with the Lichtenstein technique could not deliver sufficient evidence to determine the greater effectiveness of one over the other technique [11- 13].
There are still 4 main impacting factors affecting hernia repair results:
1. Mesh material and network design;
2. Mesh fixation
3. Tissue healing and regeneration
Surgical technique Mesh
Material and design: Theodor Bill Roth in 1890 firstly suggested that the ideal way to repair hernias was to use a prosthetic material to close the hernia defect [14]. Many materials were tried, but all failed due to complications mostly infections and rejections [15]. Multifilament common sutures were cotton and silk, which certainly contributed to the failure of the mesh procedure [16]. In 1955, Francis Usher after several disappointing attempts with different materials started using a new material, the polyolefin (Marlex) and developed a woven mesh [17]. The optimal tissue growth through its large pores was the main difference when compared to previous materials. In the days following surgery he noticed improved mesh incorporation and that fibroblast activity and collagen production progressed suitably with less giant cells [18]. In 1958, Usher published a new surgical technique using a knitted polypropylene mesh which could be autoclaved and also more rapidly incorporated by tissue [1]. Lichtenstein, 30 years later, popularized the technique as “tensionfree hernioplasty” for inguinal hernia [19].
In recent decades’ researchers have investigated various materials to optimise this aspect of mesh repair surgery. The fundamental material characteristics should be biocompatibility, resistance to infection, maintenance of adequate long-term tensile strength, rapid incorporation into the host tissue, sufficient flexibility and noncarcinogenic response [6, 20]. Poor biocompatibility of the material has proven to be a strong contributing factor in the rejection of the prosthetic material and abnormal scar tissue formation induced by an uncontrolled immunological response.
When a surgical mesh is implanted without having suitable biocompatibility (due to the material nature or its structural design) the body responds by encapsulating the foreign body, leading to the formation of a stiff scar. The compromised integration process generates pain, hernia recurrence or rejection of the mesh [21]. Currently, most of the surgical meshes are chemically and physically biocompatible, non- toxic, and non-immunogenic [1]. However, none of them is biologically inactive and this may variably impact the mesh suitability in the host tissue and the effective process of mesh integration [22].
Mesh integration, biology: Implantation of the mesh is quickly followed by an extraordinarily complex series of events that mark the initiation of the healing process. It is essential to look into the process of inflammation and its effect on the wound healing to understand the biology that supports the mesh integration process [23]. In general, the introduction of foreign material into the body triggers a response characterized by one of three stereotypical reactions: (1) destruction or lyses, (2) integration or tolerance, (3) rejection [1]. When a nonbiocompatible material is inserted into our body, the immune system recognizes it as extraneous material and therefore attempts to destroy or reject it [24]. When the implant is biocompatible a process of integration begins, this has four main stages.
First stage: The Acute Inflammation: The first biological response of the injured site starts with the coagulation of proteins around the prosthetic implant [25]. The Coagulum is composed of albumin, fibrinogen, plasminogen, complement factors and immunoglobulins [26]. Platelets adhere to these proteins activating the classical and alternative complement pathways, especially generating factors C3a and C5a (chemotactic factors for inflammatory cells) that convey polymorphonucleocytes or neutrophils (PMNs), fibroblasts, smooth muscle cells and macrophages to the wound area in an ordered sequence [27].
The chemotactic function is defined as “the movement of cells towards a preferred migration site triggered by a chemical stimulus”. During this first stage or acute phase of inflammation, migratory PMNs phagocyte microorganisms and necrotic material. When exhausted PMNs die and release their cytoplasmic and granular contents near the mesh, this may cause an additional inflammatory response [28]. If the acute inflammatory response fails to eliminate the cause of injury and to restore injured tissue to normal physiology, this condition could develop into a state of chronic inflammation.
Second stage: Chronic Inflammation: In this stage, monocytes that migrated to the wound site during the previous phase differentiate into macrophages. In addition to macrophages, other primary cellular components such as plasma cells and lymphocytes actively contribute to the advanced inflammatory response. Macrophages start the phagocytosis of dead cells, necrotic tissue and consume foreign bodies and generally prepare the way for fibroblasts settlement [29].
Third stage: Foreign Body Reaction: This kind of chronic inflammation is related to an implanted medical device or biomaterial. In response to the presence of large indigestible foreign bodies, macrophages fuse into a foreign body giant cell in the attempt to seal the extraneous material in an epithelioid granuloma [30-32]. Foreign body reaction is a complex defence response involving: foreign body giant cells, fibroblasts, and angiogenesis formation in variable amounts depending on the nature, form and structure of the implanted material [30].
Fourth stage: Scar Formation: This is characterized by the replacement of damaged tissue with fibroblasts and other cell lineages; these produce the extracellular matrix (EMC) and generate the scar. Wound healing and scar formation can be affected by persistent inflammation and the severity of the primary injury [33]. Fibroblasts are the cells that mediate the wound healing progression. These cells enter the wound site two to five days after the surgery, typically once the acute inflammatory response has receded. Fibroblasts proliferate at the wound site, reaching peak levels after one to two weeks. The main function of fibroblasts is to synthesize extracellular matrix (EMC) with collagen to regenerate the connective tissue. The EMC is itself involved in the regulation of inflammation, angiogenesis and connective tissue regeneration through the collagen matrix laid down by fibroblasts together with local GFs [24].
Main GFs involved in the game: Below are some of the most important GFs playing different roles in mesh integration and tissue regeneration. Platelet derived growth factor PDGF A-B supports smooth muscle cell and fibroblast proliferation. Fibroblast growth factor FGF-2 produced by a number of cells (endothelial, smooth muscle and fibroblast cells) is a potent activator for smooth muscle cells, endothelial cells and fibroblasts. Transforming growth factor β- TGF β is secreted by many different cells among these macrophages and PMNs. TGF β, promotes the production of fibroblasts and monocyte activation.
Insulin-like growth factor IGF made by platelets and fibroblasts is a potent chemotactic for endothelial cells. Epidermal growth factor EGF produced by platelets and macrophages promotes the production of extracellular matrix and collagen formation playing a significant role in the hernia repair. Vascular endothelial growth factor VEGF generates new blood vessels in the site of injury increasing the local blood supply [34-37].
The key role of fibroblasts: Fibroblastic activity peaks around two weeks’ post-surgery, usually on the 8th day for the intra-peritoneal access and on the 10th day for the extra-peritoneal way. The optimum amount (density) of fibroblasts needed for the successful mesh integration is achieved approximately two weeks after surgery [38]. Collagen is the principal biomechanical component of connective tissue, it provides strength and acts as a scaffold in the forms of type I, II and III [38].
A normal fibroblastic biological response leads to optimal synthesis of the connective tissue. Primarily immature, frail collagen type III is synthesized and excreted by fibroblasts as a monomeric form into the extracellular space where it polymerises into an insoluble helical structure. A fragile collagen network is produced for around the first 21 days, and then there is a modification in the ratio of collagen type III and I. The collagen type III reduces and the type I, stronger and stable, arises. The mechanical strength increases progressively until 6 months after surgery [38].
Therefore, the quality of connective tissue is significantly influenced by the collagen ratio type I/III and its contribution to making up the ECM. An altered Type I/III collagen ratio results in decreased tensile strength and mechanical stability. Thus, the alterations of collagen subtypes play a central role in the pathophysiology of hernia repair and mesh integration [39-41].
Recent Literature highlights the responsibility of enzymes like Matrix Metallo-Proteinases, MMPs and the lack of their inhibitors Tissue Inhibitors of Metalloproteinase, TIMPS to be the possible cause for the altered ratio of collagen subtypes. This modification has been quite often found in the trasversalis fascia of patients with direct inguinal hernia [42,43].
The main function of these enzymes is to degrade and expedite the turnover of the extracellular matrix (EMC) by acting on certain types of collagen and elastin [44]. MMP-1 and MMP-13 are the principal matrix enzymes responsible for the type I, II, III collagen turn over [45]. Therefore, the alterations in MMP-1 and MMP-13 protein expressions could have a role in the derangement of the ratio type I/III collagen [38]. MMP-2 and MMP-9, derived from neutrophils, play an important role in degrading collagen types IV and V as well as elastin, fibronectin, and other EMC components. A direct correlation between the altered expression of MMP-2 and MMP-9 with the diminished level of TIMP- 1 and TIMP-2 was observed in inguinal hernia patients [46,47].
Excessive fibroblasts and associated hyperactivity in the wound area will prolong the inflammatory phase resulting in increasing fibrosis. This will compromise the optimal synthesis of collagen and thereby the prosthesis integration. Prolonged inflammation can jeopardize the mesh integration and this can cause contraction and shrinkage of the mesh, eventually resulting in fibrosis, adhesions and fistulas. This can lead to prosthesis rejection [48,49].
Optimising conditions for the first two weeks’ post-surgery appears to be essential for the successful incorporation of the mesh and to produce healthy connective tissue. After 12 weeks the process is considered more stable and the overall strength of the scar increases gradually up to six months. The final result is a relatively less elastic tissue that has only 70–80% of the strength of the native connective tissue [50]. A non-absorbable prosthesis fully incorporated within newly formed collagen will help to optimise overall strength [38]. The fibrotic reaction generated by the body when a mesh is introduced is governed by the chemical nature of the material and its physical characteristics such as the filament structure and pore size [1].
Mesh Chemical and Physical Properties
Properties and design of the mesh influence the immune reaction, wound healing and integration [51].
(a) Elasticity and Tensile Strength: Either deterioration of the tensile strength of the mesh or poor flexibility or a strained mesh could lead to hernia recurrence [1].
(b)Pore Size: Porosity plays a key role in the reaction of the tissue to the prostheses. Macro porous meshes have been found to facilitate the colonization of macrophages, fibroblasts and collagen fibres formation. Large pores expedite easy infiltration of PMNs, monocytes and lymphocytes cells, providing protection from infection. Fibroblasts produce the new collagen fibres that are the scaffold for building up the EMC and facilitating mesh integration [52].
(c)Weight (density): The lightweight mesh is composed of thin filaments with large pores, generally larger than 1 mm. Lightweight meshes contain less material so reduce the impact of the foreign body reaction. A weaker immune-inflammatory response may result in better tissue incorporation [53].
(d)Structure: Surgical meshes are made using monofilament or multifilament systems. A surgical mesh formed of monofilament fibres provides good tissue reinforcement but is stiff and less pliable. A surgical mesh formed of multifilament yarns is soft and malleable. However, multifilament fibres mesh tends to retain infectious and inflammatory substances, increasing the erosion rates by 20–30% and increasing the risk of recurrence [54].
(f) Material Absorption: Surgical meshes can be made with absorbable or non-absorbable material. Non-absorbable meshes have good mechanical qualities; they are intra-operatively easy to shape and have long-term stability. However, mesh deterioration and distortion: stiffness, erosion, shrinking and adhesions have been widely reported to promote recurrence in mid-long term.
Absorbable meshes were developed to reduce these long-term complications. These meshes promote regulated postoperative fibroblast colonisation. Nevertheless, after mesh absorption is completed, the resulting scar tissue may be too weak and insufficient to provide required support resulting in increased hernia recurrence rate. For this reason, mixed meshes made with both absorbable and non-absorbable material have been introduced [1].
Commercially available surgical meshes: The “ideal” mesh should be suturable to be held in place, resistant to straining and loading under biaxial tension (eg. defecation or lifting actions) especially during the first 3 months’ postoperative period. This prosthetic implant should promote a fast and organized integration process supporting tissue regeneration together with minimal inflammatory reaction [2].
There are over 70 meshes commercially available for hernia repair, each attempt to match the “ideal” mesh characteristics. They are classified according to the composition or type of material as: (1) first generation (synthetic non-absorbable prosthesis), (2) second generation (mixed or composite prosthesis), and (3) third generation (biological prosthesis) [1,38]. For inguinal hernia surgery the most recommended are the macro porous, non- absorbable, monofilament, lightweight soft mesh. Polypropylene has been the material of choice and several companies produce in different shapes and at a range of prices.
Nanofibers prospective: Nanofibers are fibers with diameters in the nanometre range; they have found increasing scaffolding application in Tissue engineering (TE) [55]. They have been made from a variety of materials and their use explored extensively in the last decade. Specifically, nanofiber scaffolds applied in tissue regeneration, mimicking the extracellular matrix, (ECM) may provide advanced means to control MSCs migration, adhesion, proliferation, and differentiation.
For this reason, TE and nanofibers provide new perspectives for the design and production of surgical meshes that retain material strength, increase biocompatibility and promote better integration. These also open new options in laboratory research and experimental models to explore the implementation of nanofiber systems and effectively mimic the ECM [56].
Some nanofiber structures present several advantages. The manufacture of bio-mimetic scaffolds allows precise control over the geometry and microstructure of the mesh. These physical and mechanical properties make nanofiber systems suitable for new biomaterials to be used in wound care and scaffolding for tissue regeneration. The sophisticated microporous structure and modelling 3D microenvironment provide a more specific surface for cell attachment and for excellent cells–biomaterial contact [57].
Mesh Fixation
Mesh fixation is a controversial area in inguinal hernia surgery. The most common methods used to fix the hernia mesh are stitches, fibrin and cyanoacrylate glues. A minority of surgeons suggest for a non-fixation technique (mostly in TEP); however, reports about the rate of recurrence are incomplete and controversial.
Stitch fixation: The Lichtenstein technique is currently the most popular technique to repair unilateral primary groin hernias [19]. In the Lichtenstein technique the mesh fixation is provided by a Prolene (polypropylene) overrunning or single stitch suture to sew the mesh firmly to the surrounding structures: the pubic tubercle, inguinal ligament and muscles.
Some surgeons opt for absorbable stitch material such as polyglycolic acid (Vicryl) in the hope of reducing the risk of longterm nerve entrapment in the suture line. Lichtenstein technique has a generally good reported outcome in Literature: easy to perform, low morbidity and good long-term results [58]. Nonetheless, several recent articles showed high incidence of chronic inguinal pain, with an average incidence of 12%, and sometimes reported as high as 53% [59-67].
Many studies considered chronic postoperative pain as a surgical primary outcome but only a few of them evaluated the social impact of post-hernia repair chronic pain. This chronic groin pain has been reported to affect the soci al and work life of up to 6% of patients [59,61,64,66].
Specifically, in the Lichtenstein operation, chronic groin pain can be due to nerve entrapment in the suture either in the scar tissue or neuroma development, inflammation of the periosteum of the pubic tubercle (traditionally taken into the first stitch), and persistent inflammation with foreign body over-reaction to the mesh [68-69].
Lichtenstein technique has been always described as a “tension free technique”. However, stitches do generate tension and stiffness in the inguinal area particularly during muscle activity. This tension may cause postoperative mechanical pain, persistent inflammation and delay in recovery time.
Glue sealant
To avoid the above problems and reduce the risk of chronic pain, different methods of mesh fixation have been considered and most of all tissue-compatible glues. The goal is to provide a suture-less fixation.
The ideal adhesive material should be:
a. Biocompatible
b. Cheap
c. Easy to store and use.
The advantages of glue fixation are reduced postoperative acute and chronic pain (due to the nerve entrapment syndrome), improved haemostasis, and a faster operation. There is no report in Literature of any difference in recurrence when comparing glue with stitch fixation.
Fibrin glue: The main components of Fibrin glues are concentrated fibrinogen, thrombin, and calcium chloride, thus duplicating the final stage of the coagulation cascade. Fibrin acts as a haemostatic barrier, adheres to the surrounding tissue, and operates as a scaffold for migrating fibroblasts [70]. Fibrin glues are used as a tissue adhesive for a variety of surgical procedures. The main advantages of fibrin glues are tissue compatibility, biodegradability, and efficacy when applied to wet surfaces. Potential contamination by transmissible blood-borne pathogens has been criticised by some authors [71]. Fibrin glue has given very good results in tension free mesh fixation both in open and laparoscopic approaches [72- 74]. Nonetheless, it does not fulfil the requirements (b) and (c) because it is expensive and difficult to store and to prepare for the surgical application. Further, fibrin glue can potentially transmit blood-related infections [75].
Cyanoacrylates (CAs) glue: Some surgeons consider the longlateral- chain cyanoacrylates the best choice for mesh fixation in open mesh repair for inguinal hernia. CAs is biocompatible, inexpensive and easy to store and use [75]. CAs is an efficient way to seal the mesh to the nearby tissue and works as haemostatic although does not provide a scaffold or facilitate tissue regeneration.
The main problem of the CAs has been recognised in the general increase of macrophage response when compared to absorbable sutures [76]. However, this excessive inflammatory reaction induced by the CAs does not seem, in experimental models, to alter significantly the collagen maturation process or delay the mesh integration, which seems very similar to absorbable sutures [76].
In addition, there are no published studies that differentiate M1 macrophages, which promote inflammation and tissue injury, from M2 macrophages, which act to resolve inflammation and promote tissue reconstruction. Therefore, some of these cells identified in preclinical studies may be of the reparative type [76].
Platelet rich fibrin – PRF
The first platelet concentrate, platelet-rich plasma (PRP) was introduced by Marx et al. in 1998. PRP has been used with bone grafts in the attempt to repair osseous defects in dentistry, in maxillofacial/ ENT-surgery and for aesthetic and orthopaedics purposes [77,78].
PRP has been also used to accelerate soft tissue regeneration, including the facilitation of gingival, dermal and skin wound healing. PRP is also used as an injectable therapy for osteoarthritis [78,79].
The disadvantages of using PRP are the lack of uniformity in its preparation methods, the use of bovine thrombin for activation and, most of all, the inadequate period of stimulation of the target tissue. Rapid diffusion of PRP severely limits its ability to stimulate local MSCs [80].
Platelet rich fibrin (PRF) has been described as a secondgeneration autologous platelet concentrate because it does not require any biochemical additives like anticoagulants or bovine thrombin for fibrin polymerisation.
However, PRF biological properties are rather different from PRP and cannot be considered a PRP development but rather a different bio stimulator. The preparation of PRF begins with the immediate centrifugation of the patient’s venous blood collected in normal glass tubes. Choukroun et al. first described the process in 2000 [81].
PRF is an autologous biomaterial, made of a strong fibrin matrix that variably contains:
• High concentration of vital and non-vital: platelets, leucocytes and circulating MSCs
• Variable pool of cytokines
• An elevated concentration of long releasing growth factors (GFs). These include platelet derived growth factor (PDGF A-B), vascular endothelial growth factor (VEGF), transforming growth factor (TGF β -1,2), insulin-like growth factor (IGF-I), epidermal growth factor (EGF); connective tissue growth factor (CTGF); bone morphogenetic protein 2 (BMP-2) [82,83].
• An elevated concentration of fibrin, fibronectin, vitronectin, and thrombospondin.
• A variable pool of heat shock proteins HSPs (not well studied yet)
Tissue regeneration is a complex sequence mediated by an extensive range of biological- immunological events, which are regulated by various cytokines, tissue mediators and GFs. Although it is recognised that cells of different lineages generate these molecules, the details are not well known.
Platelets play a key role as an autologous source of growth factors [84,85]. Since their activation, platelets secrete multiple GFs including PDGF, TGF β-1, 2, and IGF-I [86].
Autologous platelet concentrates are widely used as a bioactive surgical component to decrease inflammation and increase the speed of the healing process. In the last few years, several different techniques have been developed to produce platelet concentrates from blood [77].
PRF production: It is inexpensive and the process is straightforward. Blood samples are collected from the patient in sterile plastic-coated tubes (8-10 ml) to be immediately treated in a centrifuge. Literature reports various protocols to centrifuge blood and thereby produce different kinds of PRF with different properties. Two well studied PRF products are A-PRF (Advanced Platelet Rich Fibrin) and L-PRF (Leucocytes Platelet Rich Fibrin). Specific PRF biological properties can be created by adjustments to the characteristics and methods of centrifugation [83].
L-PRF typically is made with 9 ml fresh blood in glass-coated plastic tubes, immediately centrifuged at 2700 rpm for 12 minutes. A-PRF is made with 10 ml fresh blood in glass tubes, immediately centrifuged at 1500 rpm for 14 minutes [83]. Centrifuge stability, vibration and the temperature developed in the tubes determine the properties and quality of the final PRF. Specifically, the centrifuge characteristics impact on the making of L-PRF clot and membrane and this in turn determines cells survival, fibrin architecture and also the GFs contained in them [83].
In comparison to the L-PRF membrane, the A-PRF clots and membranes appear much smaller, more fragile and less separated from the red blood cell layer. The original L-PRF shows a strongly polymerized thick fibrin matrix and large numbers of cells appear alive with a normal shape, including activated lymphocytes [87].
The A-PRF shows lightly polymerized slim fibrin gel and most of the visible cell bodies appear damaged or destroyed. In addition, L-PRF membranes in vitro maintain good health for 7 days and slowly release their growth factors for at least 7 days; whereas A-PRF membranes dissolve in vitro in less than 3 days and their GFs rapidly dissipate or decline [83,87].
After centrifugation, through the activation of autologous thrombin, a fibrin clot is created. Three distinct layers can be seen in the tube (Figure 1) red blood corpuscles RBCs at the bottom of the tube, platelet-poor plasma PPP on the top of the tube, and the PRF clot in the middle of the tube. The PRF clot can be removed from the tube with surgical tweezers (Figure 2). The clot by itself contains a great amount of exudate, which is rich in growth factors, and this exudate can be pressed out by gentle compression of the clot in order to obtain PRF membranes.
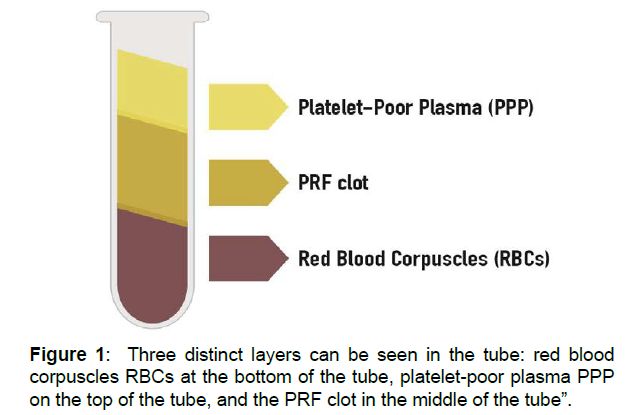
Figure 1: Three distinct layers can be seen in the tube: red blood corpuscles RBCs at the bottom of the tube, platelet-poor plasma PPP on the top of the tube, and the PRF clot in the middle of the tube”.
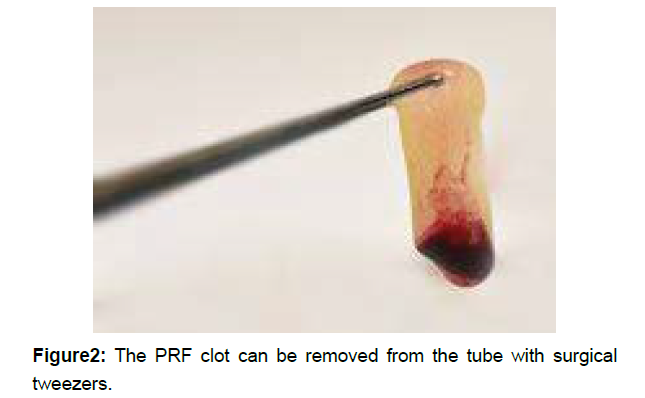
Figure 2: The PRF clot can be removed from the tube with surgical tweezers.
Serum squeezed out from the PRF clot, called hyper-acute serum, has a greater cell proliferative effect on different connective cell lineages such as bone marrow mesenchymal stem cells (MSCs), osteoblasts and chondroblasts cells [88,89]. After removing the hyper-acute serum fraction, the remaining PRF membrane is a threedimensional, adhesive, biocompatible and biodegradable scaffold. The membrane surface and ECM structure facilitate contact and cell interactions. Furthermore, PRF membranes are able to slowly release bioactive molecules that facilitate migration, adhesion and proliferation of local MSCs [77,90,91].
During centrifugation, the soluble fibrinogen contained in the plasma transforms to fibrin polymerizing to a three-dimensional structure. The activated platelets and some leukocytes are entrapped in the fibrin net. Consequently, a storage pool of growth factors is formed from platelets and leukocytes upon activation [92].
Platelets can be observed both on the surface and inside of the PRF membranes. The platelets’ GFs are widely used in soft and bone tissue regeneration; one of the better known is the PDGF, which enhances MSCs adhesion and proliferation [77].
Kardos D. et al observed that MSCs seeded onto PRF membranes readily proliferated on the surface of fresh, but even better on freeze/ thawed or freeze-dried membranes [77]. Freeze-drying seems to not impair the stability of membranes’ biofactors, the structure of fibrinogen or the clinical applicability of the PRF [93]. The advantage of producing freeze/thawed or freeze/dried membranes is their suitability for wound closing because their tensile strength can hold a suture and might be more fit for some surgical procedures. In addition, freezing the membranes at −20°C and thawing at +4°C may help to slow down the process of membrane degradation. Therefore, some Authors observed that exposing injured tissue to prolonged membrane stimulation enhances the effectiveness of tissue regeneration [77].
Although, it is interesting to observe that living cells have been found in fresh membranes only, while frozen and freeze-dried membranes contained only dead cells [94]. This tissue regeneration of freeze-dried membranes could be partly due to the HSPs concentration produced during the freezing process [95].
PRF clinical application: PRF has been successfully used for the treatment of non- responding skin ulcers including diabetic foot ulcers (DFU), pressure ulcers (PU), acute surgical wounds, and venous leg ulcers (VLUs) [96]. The beneficial effect of L-PRF membranes in the healing of chronic leg ulcers can be explained by the high concentration of platelets and leucocytes, together with the long-term release of growth factors. The progressive release of growth factors (eg. TGFß-1, TGFß-2, PDGF-AB, VEGF, EGF, IGF and CTGF), matrix glycoproteins (trombospondin-1, fibronectine and vitronectine), inflammatory regulators as cytokines and various HSPs for several days seem to be crucial to promote the healing process [97,98].
In dentistry and maxillofacial surgery, the application of PRF membrane is widespread. There are numerous described procedures including the treatment of periodontal bony defects and regeneration, ridge preservation, sinus-floor elevation, implant surgery, and the creation of the PRF bone block [99,100]. PRF increases new bone formation and has a positive effect on early bone healing [101]. Wong C. et al have applied PRF in experimental models to promoting meniscus chondroblasts proliferation in the attempt to facilitate meniscal repair [102].
In dermatology and plastic surgery have been reports of dermal fibroblasts migration and activation resulting in the increase of collagen synthesis of the skin exposed to PRF treatment [103,104].
We have recently started using L-PRF clot and its constituents PRF membranes and hyper-acute serum to fixing the mesh in inguinal hernia open mesh repair procedure. Preliminary results are very promising.
The aim of the current study has been to fix the mesh with some glue to achieve a real “free tension technique”. Therefore, avoiding stitches, tensions and tractions of tissue. PRF is less expensive than either fibrin glue or cyanoacrylate.
PRF provides similar scaffolding properties to fibrin glue and eliminates the risk of transmission of blood pathogens. Furthermore, PRF has beneficial anti-inflammatory properties as opposed to the characteristic property and problem of cyanoacrylates, the promotion of inflammatory tissue reactions. Most important, PRF exhibits both good fixation capacity and strong tissue regeneration properties. PRF minimises the local inflammatory acute response, streamlining the integration of the mesh through rapid fibroblast colonization and efficient collagen production. These elements in combination result in a faster and more effective wound healing process
Regenerative surgery technique: PRF-open mesh repair
Open mesh repair for single inguinal hernia is the most frequent procedure in general surgery.
The surgical technique should apply 3 basic principles to achieve the best clinical result: minimally invasive approach, tension-free technique and regenerative surgery principles. The open mesh repair procedure can be delivered in local or general anaesthesia. A small (5-6 cm) transverse incision on the inguinal region reduces skin tension following the natural Langer’s lines; they are linear clefts in the skin that indicate the direction and orientation of the underlying collagen fibers. A small incision generally provides a proper exposition of the inguinal region. Retractors need to be moved gently; they should have smooth and not dentate edges. Minimal manipulation is suggested to avoid any stretching and shredding of tissue. Tissue needs to be sharply cut following a constant dissection line, recognising any different tissue layers to perform those minimal dissections necessary for completing the operation. Haemostasis needs to be checked step by step.
After the sac has been isolated and content examined, it will be repositioned into the abdomen and the internal inguinal ring refashioned using polyglactin (vicryl) 2-0 stitches. In case of direct hernia, the trasversalis fascia needs to be approximated with the same suture material. Mesh placement. In preparing a suitable space to position the mesh, it is important to minimise the detachment of tissues and in particular to respect as much as possible the nerves that cross the area. The choice of an appropriate size, soft, light, macroporus, monofilament, polypropylene mesh is fundamental, as is the fixation method.
Mesh fixation. In our preliminary experience, we used L-PRF clot with both components: membrane and hyper-acute serum to fixing the mesh and secure a real tension free technique.
Only two single stitches have been applied to the mesh: one in the tubercle area (never catching the tubercle!) and another one to close the mesh tails. Vicryl 2-0 absorbable is always used to reduce risks of chronic nerve entrapment syndrome.
Anterior fascia closure. We suggest suturing the fascia below the spermatic cord using vicryl 2-0 stitches. This recommendation is to reduce the empty space and press the mesh between the trasversalis (posterior) and anterior fascia, ensuring that the spermatic cord in not the way.
The fascia, sutured with absorbable (vicryl 2-0) stitches, places and compresses the mesh in the right space reducing the postoperative collection, indeed facilitating the integration process.
These basic principles reduce oedema, collection and postoperative acute inflammation. These are the main factors that hinder the integration process and trigger postoperative pain.
In particular, PRF combines the benefits of fibrin glue sealant with the ability to streamline the integration of the mesh by optimising connective tissue regeneration. Moreover, PRF regenerative capacities substantially prevent chronic fibrotic inflammation, mesh retraction, hard and painful scars and chronic nerve entrapment syndrome.
Discussion
The aim of this article is to introduce regenerative surgery standards to promote optimal tissue regeneration pathways for the most common surgical procedure in general surgery: the open mesh repair for inguinal hernia. The clinical perspective of the PRF-open mesh repair is the reduction of postoperative pain, to accelerate the patient’s recovery, prevent the chronic inguinal pain, reduce the rate of recurrence and reduce financial costs of the operation. Our preliminary PRF-open mesh repair experience and data will be published soon. Accurate surgical technique, tissue regeneration principles and the right material choices are basic to reach the goal.
Lichtenstein technique promotes a rigid fixation of the mesh, recommending non- absorbable overrunning stitches (Prolene) to sew the mesh to the inguinal ligament and surrounding muscles structures; this generates tension, inflammation and pain.
In addition, the risk that a permanent suture would trap a nerve is reasonably high (average 11-12% in Literature) causing chronic inguinal pain, which quite often requires local infiltration or unreliable nerve-lysis operations.
These risks are significantly reduced by fixing the mesh to the trasversalis (posterior) fascia with the L-PRF and making a real “tension-free” technique.
In 2009, De Hingh I.H.J.T et al, described the use of autologus P-RFS (Platelet-Rich Fibrin Sealant) on 22 patients. The aim was fixing the mesh replacing the human/bovine fibrin glue. The advantages claimed focused on the P-RFS haemostatic and antibacterial effects. The preparation of the blood required a large amount of patient’s own blood (120 ml) and stored into a designated preparation unit containing sodium citrate for anticoagulation. Afterwards, the container was placed in the centrifuge for 23 min to produce an average of 6 ml of P-RFS. With a spray applicator, the P-RFS was applied along the ligament and the medial and cranial side of the mesh [105].
In our preliminary experience, L-PRF shows some additional and essential clinical improvements over previous practice: 1) satisfactory fixation of the mesh; 2) superior haemostasis; 3) less local inflammation; 4) avoidance of nerve entrapment; 5) streamlining the mesh integration process and tissue regeneration. Long term clinical trials may show that improved integration leads to a reduction in recurrence.
The L-PRF is an autologous platelet rich fibrin centrifuge product. The centrifuge characteristics and centrifugation protocols impact significantly on the characteristics of PRF. The centrifugation protocol we have been using enables production of L-PRF with good glue and scaffolding performance and also with tissue regeneration properties. Preparation procedure will be described in the material and method section of the upcoming article. Compared with fibrin glue, the cost of the PRF open mesh repair is substantially lower because the fixation glue is made with the patient’s own blood.
Conclusion
PRF-open mesh repair is a physiologic and genuinely “tensionfree technique” that follows sound regenerative surgery principles. The surgical technique, material choice, biological method of fixation and the regenerative properties of PRF, all described above, minimise wound site inflammation and assist correct integration of the mesh. Postoperative advantages: less pain and shorter time of recovery are clear short-term benefits. Mid-long term benefits: lower incidence of chronic pain due to a correct process of wound healing and minimizing the risk of nerve entrapment. A reduction of recurrence rate may be confirmed by future randomized trials.
Conflicts of interest
The author declares that he does not have financial or nonfinancial competing interests.
Acknowledgments
The author sincerely compliments Ms Jordana Lyden-Swift for drafting images. The author is grateful to Thomas Richard Swift for the linguistic revision of the manuscript and his passionate interest in the Regenerative Surgery field
References
- Elías-Zúniga, Robert G, Lozano K (2017) Past, Present and Future of Surgical Meshes: A Review. Membranes(Basel).7:47
- Bendavid R, Abrahamson J, Arregui ME, Flament JB, Phillips EH (2001) Abdominal Wall Hernias: Principles and Management
- Heniford BT (2015) Hernia Handbook. 1st ed. Carolinas HealthCare System; Charlotte
- Kingsnorth A (2004) Treating inguinal hernias: Open mesh Lichtenstein operation is preferred over laparoscopy. BMJ.328:59–60.
- Li X, Kruger JA, Jor JW, Wong V, Dietz HP, et al (2014) Characterizing the ex vivo mechanical properties of synthetic polypropylene surgical mesh. J. Mech Behav Biomed Mater.37:48–55.
- Pandit AS, Henry JA (2004) Design of surgical meshes - An engineering perspective. Technol Heal Care.12:51–65.
- EU Hernia (2002) Trialists Collaboration Repair of groin hernia with synthetic mesh: Meta-analysis of randomized. Ann Surg. 235:322–332
- Köckerling F, Koch A, Lorenz R, Schug-Pass C, Stechemesser B, et al.(2015)How long do we need to follow-up our hernia patients to find the real recurrence rate? Front Surg. 2:24.
- HerniaSurge Group (2018) International guidelines for groin hernia management. Hernia. 22:1-165
- Köckerling F, Simons MP (2018) Current Concepts of Inguinal Hernia Repair. Visc Med. 34:145-150
- Koning GG, Wetterslev J, van Laarhoven CJ, Keus F (2013) The totally extraperitoneal method versus Lichtenstein's technique for inguinal hernia repair: a systematic review with meta-analyses and trial sequential analyses of randomized clinical trials. PLoS One. 8:52599.
- Bobo Z, Nan W, Qin Q, Tao W, Jianquo L, et al. (2014) Meta-analysis of randomized controlled trials comparing Lichtenstein and totally extraperitoneal laparoscopic hernioplasty in treatment of inguinal hernias. J Surg Res. 192:409-420
- Scheuermann U, Niebisch S, Lyros O, Jansen-Winkeln B, Gockel I (2017) Transabdominal preperitoneal (TAPP) versus Lichtenstein operation for primary inguinal hernia - a systematic review and meta-analysis of randomized controlled trials. BMC Surg. 17: 55
- Billroth T (1924) In: The Medical Sciences in the German Universities: A Study in the History of Civilization. Welch W.H., editor. Macmillan; New York, NY, USA
- Chowbey P (2012) Endoscopic Repair of Abdominal Wall Hernias. 2nd ed. Byword Books; Delhi, India
- LeBlanc KA (2003) Laparoscopic Hernia Surgery an Operative Guide. 1st ed. CRC Press; New Orleans, LA, USA
- Usher FC, Hill JR, Ochsner JL (1959) Hernia repair with Marlex mesh. A comparison of techniques. Surgery. 46:718–728.
- Klinge U, Klosterhalfen B, Birkenhauer V, Junge K, Conze J, et al. (2002) Impact of polymer pore size on the interface scar formation in a rat model. Surg. Res. 103:208–214
- Lichtenstein IL, Shulman AG, Amid PK (1989) The tension free hernioplasty. Am J Surg. 157:188–193
- Melero Correas H (2008) Master Thesis. Universitat Politècnica de Catalunya; Barcelona, Spain: Mechanical Characterization of Surgical Meshes for the Repair of Abdominal Hernias.
- Hawn MT, Gray SH, Snyder CW, Graham LA, Vick CC, et al. (2011) Predictors of mesh explantation after incisional hernia repair. Am. J. Surg. 202:28–33.
- Schumpelick V, Fitzgibbons R.J (2010) Hernia Repair Sequelae.1st ed. Springer; Berlin/Heidelberg, Germany
- Bendavid R (1994) Prostheses and Abdominal Wall Hernias. 1st ed. R.G. Landes Co.Austin, TX, USA
- Anderson J.M (2001) Biological Response to Materials. Annu. Rev.Mater. Res. 31:81–110.
- Tang L, Ugarova TP, Plow EF, Eaton JW (1996) Molecular determinates of acute inflammatory response to biomaterials. J. Clin. Invest.; 97:1329–13234.
- Busuttil SJ, Ploplis VA, Castellino FJ, Tang L (2004) A central role for plasminogen in the inflammatory response to biomaterials. J. Thromb. Haemost. 2:1798–1805
- Earle DB, Mark LA (2008) Prosthetic Material in Inguinal Hernia Repair: How Do I Choose? Surg. Clin. North Am.88:179–201.
- Schaechter M (2009) Encyclopedia of Microbiology. 3rd ed. Academic Press; Cambridge, MA, USA
- Greenberg JA, Clark RM (2009) Advances in suture material for obstetric and gynecologic surgery. Rev. Obstet. Gynecol.2:146–158
- Anderson JM, Rodriguez A, Chang DT (2008) Foreign Body Reaction to Biomaterials. Semin. Immunol. 20:86–100.
- Murch AR, Grounds AD, Marshall CA, Papadimitriou JM (1982) Direct evidence that inflammatory multinucleate giant cells form by fusion. J Pathol.137: 177– 180
- Woloson SK, Greisler HP, Bendavid R, Abrahamson J ( 2001) Biochemistry, immunology, and tissue response to prosthetic material. In:EditorsAbdominal wall hernias. Principles and management. New York: Springer-Verlag: 201–207.
- Chu C, Von Fraunhofer JA, Greisler HP (1997) Wound Closure Biomaterials and Devices. 1st ed. CRC Press LLC; Boca Raton, FL, USA
- Bennett NT, Schultz GS (1993) Growth factors and wound healing: biochemical properties of growth factors and their receptors. Am J Surg. 165: 728–737
- Greisler HP, Ellinger J, Henderson HC (1991) The effects of an atherogenic diet on macrophage/biomaterial interaction. J Vasc Surg.; 14: 10.
- Petsikas D, Cziperle DL, Lam TM (1991) Dacron-induced TGF-b release from macrophages: effects on graft healing. Surg Forum. 42: 326–328.
- Bellon JM, Bujan J, Contreras L, Hernando A (1995) Integrations of biomaterials implanted into abdominal wall: process of Scar formation and macrophage response. Biomaterials.16: 381–387.
- Elango S, Perumalsamy S, Ramachandran K, Vadodaria K (2017) Mesh materials and hernia repair. Biomedicine (Taipei). 7: 16.
- Junge K, Rosch R, Klinge U, Schwab R, Peiper C, et al (2006) Risk factors related to recurrence in inguinal hernia repair: a retrospective analysis. Hernia.10: 309–315.
- Si Z, Bhardwaj R, Rosch R, Mertens PR, Klosterhalfen B, et al (2002) Impaired balance of type I and type III procollagen mRNA in cultured fibroblasts of patients with incisional hernia.Surgery.131: 324–331
- Rangaraj A, Harding K, Leaper D (2011) Role of collagen in wound management. Wounds. 7: 54–63.
- Abci I, Bilgi S, Altan A (2005) Role of TIMP-2 in Fascia Transversalis on Development of Inguinal Hernias. J Invest Surg.18: 123–128
- Guillen-Marti J, Diaz R, Quiles MT, Lopez-Cano M, Vilallonga R, et al (2009) MMPs/TIMPs and inflammatory signalling de-regulation in human incisional hernia tissues. J Cell Mol Med.13(11-12), 4432–4443
- Bellön JM, Bajo A, Ga-Honduvilla N, Gimeno MJ, Pascual G, et al (2001) Fibroblasts From the Transversalis Fascia of Young Patients With Direct Inguinal Hernias Show Constitutive MMP-2 Overexpression. Ann Surg.; 233: 287– 291
- Donahue TR, Hiatt JR, Busuttil RW (2006) Collagenase and surgical disease.Hernia.10: 478–485.
- Ashcroft GS, Herrick SE, Tarnuzzer RW (1997) Human ageing impairs injury-induced in vivo expression of tissue inhibitor of matrix metalloproteinases (TIMP)-1 and -2 proteins and mRNA. J Pathol.183:169–176
- Lau H, Fang C, Yuen WK, Patil NG (2007) Risk factors for inguinal hernia in adult males: A case-control study. Surgery.141: 262–266
- Klinge U, Zheng H, Si ZY, Schumpelick V, Bhardwaj R, et al. (1999) Synthesis of type I and III collagen, expression of fibronectin and matrix metalloproteinases-1 and -13 in hernial sac of patients with inguinal hernia. Int J Surg Investig.1: 219–227.
- Bendavid R (2004) The unified theory of hernia formation. Hernia.8: 171–176
- Earle DB, Mark LA (2008) Prosthetic Material in Inguinal Hernia Repair: How Do I Choose? Surg. Clin. North Am.88:179–201
- Brown CN, Finch JG (2010) Which mesh for hernia repair? Ann. R. Coll. Surg. Engl.92:272–278.
- Zogbi L (2008) The Use of Biomaterials to Treat Abdominal Hernias.InTech.18: 359–382
- Bilsel Y, Abci I (2012) The search for ideal hernia repair; mesh materials and types. Int. J. Surg.10:317–321.
- Winters JC, Fitzgerald MP, Barber MD (2006) The use of synthetic mesh in female pelvic reconstructive surgery. BJU Int. 98:70–76
- Vasita R, Katti DS (2006) Nanofibers and their applications in tissue engineering. Int. J. Nanomedicine.; 1:15–30.
- Popat K, Brown P, Stevens K (2010) Nanotechnology in Tissue Engineering and Regenerative Medicine & Nanofibers and Nanotechnology in Textiles 1st ed. CRC Press; Boca Raton, FL, USA
- Halm JA (2005) Thesis. Erasmus University Rotterdam; Rotterdam, The Netherlands: Oct. Experimental and Clinical Approaches to Hernia Treatment and Prevention.
- Amid PK, Lichtenstein IL (1998) Long-term results and current status of the Lichtenstein open tension-free hernioplasty. Hernia. 2: 89-94.
- Bay-Nielsen M, Kehlet H, Strand L, Malmstrom J, Andersen FH, et al (2001) Quality assessment of 26,304 herniorrhaphies in Denmark: a prospective nationwide study. Lancet. 6358: 1124-1128
- Erhan Y, Erhan E, Aydede H, Mercan M, Tok D (2008) Chronic pain after Lichtestein and preperitoneal (posterior) hernia repair. Can J Surg; 51: 383-387.
- Franneby U, Sandblom G, Nordin P, Nyern O, Gunnarson U (2006) Risk factors for long- term pain after hernia surgery. Ann Surg; 244: 212-219
- Kumar S, Wilson RG, Nixon SJ, Macintyre IM (2002) Chronic pain after laparoscopic and open mesh repair of groin hernia. Br J Surg; 89: 1476-1479
- Paajanen H (2002) Do absorbable mesh sutures cause less chronic pain than nonabsorbable sutures after Lichtenstein inguinal herniorraphy? Hernia; 6: 26-28.
- Poobalan AS, Bruce J, King PM, Chambers WA, Krukowski ZH (2001) Chronic pain and quality of life following open inguinal hernia repair. Br J Surg; 88: 1122- 1126
- Poobalan AS, Bruce J, Smith WC, King PM, Krukowski ZH (2003) A review of chronic pain after inguinal herniorraphy. Clin J Pain.19: 48-54
- Kehlet H (2008) Chronic pain after groin hernia repair. Br J Surg; 95: 135-136.
- Bay-Nielsen M, Nilsson E, Nordin P, Kehlet H (2004) Chronic pain after open mesh and sutured repair of indirect inguinal hernia in young males. Br J Surg. 91:1372-1376
- Helbling C, Schlumpf R (2003) Sutureless Lichtenstein: first results of a prospective randomised clinical trial. Hernia. 7: 80-84
- Klosterhalfen B, Klinge U, Hermanns B, Schumpelick V (2000) Pathologie traditioneller chirurgischer Netze zur Hernienreparation nach langzeitimplantation in Menschen. Chirurg .71: 43-51
- Brennan M (1991) Fibrine Glue.Blood Rev 5:240-244
- Canonico S (2003) The use of human fibrin glue in the surgical operations. Acta Biomed. 2:21-5
- Campanelli G, Champault G, Pascual MH, Hoeferlin A, Kingsnorth A (2008) Randomized controlled blinded trial of Tissucol/Tisseel for mesh fixation in patients undergoing Lichtenstein technique for primary inguinal hernia repair: rationale and study design of the TIMELI trial. Hernia.12: 159-165.
- Lovisetto F, Zonta S, Rota E, Mazzilli M, Bardone M (2007) Use of human fibril glue (Tissucol) versus staples for mesh fixation in laparoscopic transabdominal preperitoneal hernioplasty: a prospective, randomized study. Ann Surg. 245: 222-231
- Schwab R, Schumacher O, Junge K, Binnebosel M, Klinge U (2006) Fibrin sealant for mesh fixation in Lichtestein repair: biomechanical analysis of different techniques. Hernia; 11:139-145.
- Tebala GD, Tognoni V, Tristram Z, Macciocchi F, Innocenti P (2015) Cyanoacrylate glue versus suture fixation of mesh in inguinal hernia open repair a randomized controlled clinical trial. Gastroenterol Hepatol.2
- Pascual G, Mesa-Ciller C, Rodríguez M, Pérez-Köhler B (2018) Pre-clinical assay of the tissue integration and mechanical adhesion of several types of cyanoacrylate adhesives in the fixation of lightweight polypropylene meshes for abdominal hernia repair. Plose one.13:e0206515
- Kardos D, Hornyák I, Simon M, Hinsenkamp A, Marschall B (2018) Biological and Mechanical Properties of Platelet-Rich Fibrin Membranes after Thermal Manipulation and Preparation in a Single-Syringe Closed System. Int J Mol Sci.19: 3433
- Kobayashi E, Fujioka-Kobayashi M, Sculean A, Chappuis V (2017) Effects of platelet rich plasma (PRP) on human gingival fibroblast, osteoblast and periodontal ligament cell behaviour. BMC Oral Health.17:91
- Chicharro-Alcantara D, Rubio-Zaragoza M, Damia-Gimenez E, Carrillo-Poveda JM (2018) Platelet Rich Plasma: New Insights for Cutaneous Wound Healing Management. J.Funct. Biomater. 9:10
- Khiste S.V, Naik Tari R (2013) Platelet-Rich Fibrin as a Biofuel for Tissue Regeneration. ISRN Biomaterials.6
- Theys T, Van Hoylandt A, Broeckx CE, Van Gerven L (2018) Plasma-rich fibrin in neurosurgery: A feasibility study. Acta Neurochir (Wien).160: 1497-1503
- Dhurat R, Sukesh MS (2014) Principles and Methods of Preparation of Platelet-Rich Plasma: A Review and Author's Perspective. J Cutan Aesthet Surg.7: 189–197
- Doan Ehrenfest DM, Pinto NR, Pereda A, Jiménez P, Corso MD (2018) Impact of the centrifuge characteristics and centrifugation protocols on the cells, growth factors, and fibrin architecture of a leukocyte- and platelet-rich fibrin (L- PRF) clot and membrane. Platelets.29 : 171-184
- Saluja H, Dehane V, Mahindra U (2011) Platelet-Rich fibrin: A second generation platelet concentrate and a new friend of oral and maxillofacial surgeons. Ann.Maxillofac.Surg.1:53–57
- Duregger K, Gable A, Eblenkamp M (2018) Development and evaluation of a spray applicator for platelet-rich plasma. Colloids Surf. B Biointerfaces.171:214– 223
- Naik B, Karunakar P, Jayadev M, Marshal VR. (2013) Role of Platelet rich fibrin in wound healing: A critical review. J. Conserv. Dent.16:284–293
- Madurantakam P, Yoganarasimha S, Hasan FK (2015) Characterization of Leukocyte- platelet Rich Fibrin, A Novel Biomaterial. J Vis Exp.103: 53221.
- Simon M, Major B, Vácz G, Kuten O (2018) The Effects of Hyperacute Serum on the Elements of the Human Subchondral Bone Marrow Niche. Stem Cells Int:4854619
- Kuten O, Simon M, Hornyak I, De Luna-Preitschopf A (2018) The Effects of Hyperacute Serum on Adipogenesis and Cell Proliferation of Mesenchymal Stromal Cells.Tissue Eng. Part A.24:1011–1021
- Di Liddo R, Bertalot T, Borean A, Pirola I (2018) Leucocyte and Platelet-rich Fibrin: A carrier of autologous multipotent cells for regenerative medicine. J. Cell.Mol. Med.22:1840–1854.
- Aulino P, Costa A, Chiaravalloti E, Perniconi B (2015) Muscle Extracellular Matrix Scaffold Is a Multipotent Environment. Int. J. Med. Sci.12:336–340.
- Varela HA, Souza JC, Nascimento RM, Araujo RF (2019) Injectable platelet rich fibrin: Cell content, morphological, and protein characterization. Clin.Oral Investig.23:1309-1318.
- Zhang J, Qi X, Luo X, Li D, Wang H, Li T (2017) Clinical and immunohistochemical performance of lyophilized platelet-rich fibrin (Ly-PRF) on tissue regeneration. Clin. Implant Dent. Relat. Res.19:466–477
- Li Q, Reed DA, Min L, Gopinathan G, Li S (2014) Lyophilized platelet- rich fibrin (PRF) promotes craniofacial bone regeneration through Runx2. Int. J. Mol. Sci.15:8509–8525.
- Di Nicola V, Pierpaoli W (2013) Biological baseline of joint self-repair procedures. Curr Aging Sci.6
- Pinto NR, Ubilla M, Zamora Y, Del Rio V (2017) Leucocyte- and platelet-rich fibrin (L-PRF) as a regenerative medicine strategy for the treatment of refractory leg ulcers: a prospective cohort study. Platelets. 29:468-475
- Dohan Ehrenfest DM, Bielecki T, Jimbo R, Barbe G (2012) Do the fibrin architecture and leukocyte content influence the growth factor release of platelet concentrates? An evidence-based answer comparing a pure platelet-rich plasma (P-PRP) gel and a leukocyte- and platelet- rich fibrin (L-PRF). Curr Pharm Biotechnol.13:1145-1152
- Dohan Ehrenfest DM, de Peppo GM, Doglioli P, Sammartino G (2009) Slow release of growth factors and thrombospondin-1 in Choukroun’s platelet-rich fibrin (PRF): a gold standard to achieve for all surgical platelet concentrates technologies. Growth Factors.27:63-69.
- De Almeida Barros Mourao CF, Calasans-Maia MD, de Mello Machado RC (2018) The use of platelet-rich fibrin as a hemostatic material in oral soft tissues.Oral Maxillofac.Surg.22:329–333
- Bakhtiar H, Esmaeili S, Ellini M.R, Nekoofar MH (2017) Second-generation Platelet Concentrate (Platelet-rich Fibrin) as a Scaffold in Regenerative Endodontics:A Case Series. J.Endod.43:401–408.
- Miron RJ, Pikos MA (2018) Sinus Augmentation Using Platelet-Rich Fibrin With or without a Bone Graft: What Is the Consensus? Compend. Contin. Educ.Dent.39:355–361.
- Wong CC, Kuo TF, Yang TL, Tsuang YH, Lin MF (2017) Platelet-Rich Fibrin Facilitates Rabbit Meniscal Repair by Promoting Meniscocytes Proliferation, Migration, and Extracellular Matrix Synthesis. Int. J. Mol. Sci.18:1722
- Desai CB, Mahindra UR, Kini YK, Bakshi MK (2013) Use of Platelet-Rich Fibrin over Skin Wounds: Modified Secondary Intention Healing. J. Cutan. Aesthet. Surg.6:35–37
- Crisci A, Marotta G, Licito A, Serra E (2018) Use of Leukocyte Platelet (L-PRF) Rich Fibrin in Diabetic Foot Ulcer with Osteomyelitis (Three Clinical Cases Report) Diseases.6:30
- Nienhuijs SW, Overdevest EP, Scheele K, Everts PA (2009) Mesh Fixation with Autologous Platelet-Rich Fibrin Sealant in Inguinal Hernia Repair. Eur Surg Res.43:306–309.